Small Animal Clinical Services College of Veterinary Medicine, University of Florida
Gainesville, FL, USA
INTRODUCTION
Stereotactic radiosurgery (SRS) describes the application of a single high dose of radiation delivered to a stereotactically defined target volume. Radiosurgery is unique hybrid between surgery and radiotherapy and attempts to achieve surgical precision utilizing radiation. It does this by combining targeting mechanisms (stereotaxis) and beam collimation to achieve precise delivery to the target thus allowing for a single therapeutic dose to be delivered. Because of the precision of SRS more normal tissue is spared (1-3). In comparison, conventional radiation therapy relies on the differences in repair capacity between normal and pathological tissue to injure the target lesion selectively. To achieve this, conventional radiation relies on fractionated treatment programs. It also employs patient position and beam delivery to 2-4 static treatment fields.
Only a single article has been published reviewing SRS in dogs with brain tumors. Lester et al from the University of Florida described the technique using a stereotactic head ring (Brown-Roberts-Wells) (1). This technique requires the CT and the SRS to be performed on the same day because of the placement of the head ring. This method is cumbersome. Currently we employ a SRS technique which makes use of a frameless stereotactic technique using a biteplate (with fiducial array) attached to the patients maxillary dentition for cranial neoplasia (4). In the case of osteosarcoma Steinman pins are used to fix the fiducial array to the bone. This paper reviews the use of frameless stereotactic radiosurgery in dogs with cancers treated at the College of Veterinary Medicine, University of Florida.
ACQUISITION OF THE IMAGE
The procedure involves an initial CT imaging of the cancer. In the case of cancers of the head, prior to doing a CT scan, a bite plate with the fiducial array is attached to the maxillary dentition of the dog or cat. Accurate positioning is made possible by molding the bite plate to the teeth using dental impression material (Reprosil®). The bite plate and array can be removed after the CT and replaced when required for the SRS (no longer than 5 days, due to possible changes in the cancer). CT imaging is used primarily because of its accuracy. When we treat osteosarcomas, surgically placed Steinman pins are used to fix the fiducial array close to the bone.
A Cartesian system of x,y, and z vectors are used to map the target. Thus each pixel has a unique address to identify its position and each position (pixel) in the CT has an address that can relate to the fiducial array. The scan is done using 2 mm slices of the target area and surrounding structures including the bite plate area. MRI can be used, because of its superior soft tissue imaging, and is fused (image fusion) with the CT using propriety software. In the case of extracranial soft tissue cancers, the bite plate is not required; however, a CT is still done. Accuracy is achieved for these cancers by fusing an ultrasound image (US) with a CT image at the time of therapy (5). This technique utilizes a fiducial array attached to the ultrasound transducer and will be described later. On completion of the CT scan, images are sent via the internet using DICOM-3 (digital imaging communication medicine) format to a terminal with software planning situated at the Neurosurgery Department (MC Knight Brain Institute, College of Medicine, University of Florida).
MERGING THE IMAGE
A computer program automatically identifies the positioning of the fiducial array with its reflective markers in the CT image and merges the CT images with the planning software. In the case of the soft tissue tumors the dog or cat is positioned on the treatment table in the treatment room and the cancer (target) is positioned at the isocenter (center of the gantry arc) of the modified Linac using laser position devices. A LED camera in the room identifies the position of the fiducial array attached to the transducer. The tumor is then scanned using US and the position of the cancer using US is merged with the CT image.
TREATMENT PLANNING
The goals of SRS are to deliver a homogenous dose of radiation to the target and to spare vital or radiosensitive normal tissue. The ideal radiation planning would deliver 100% of the desired dose to the treatment target and none to normal tissue. This is technically not possible. In essence SRS planning tries to achieve a plan that conforms to the target as closely as possible, as defined by the radiation isodose shells (isodose lines) within the plan (3). To understand SRS planning some basic radiation physics is necessary. Radiation dose (unit=Gray) to the body is represented by isodose lines, these are lines of equal radiation dose displayed in the planning software. These lines are in fact three dimensional and take on the appearance of ellipses or spheres especially in SRS, see Figure 1. As the photon beam from the Linac (6 MV) arrives at the absorbing material (body surface) its intensity decreases exponentially due to transfer of energy to tissue.
The area of maximum delivery (Dmax) is not on the surface as one would expect but rather a distance into the tissue (variable depending on the source of radiation). The reason for this phenomenon is that photons do not deposit energy directly in the tissue but rather interact with tissue to create electrons and low-energy photons which are deposited. Depending on the type of delivery system the build up region varies in depth e.g., orthovoltage is more superficial, megavoltage (Linac) is deeper. As the photons continue past the Dmax region they become more depleted of energy which leads to a decrease in the deposited dose (falloff region). This is obviously not ideal as the Dmax does not always coincide with the target (cancer), see Figure 1. Conventional radiotherapy employs patient position, masking of vital structures and beam delivery to 2-4 static treatment fields to be able to deliver the correct dose to the target. SRS is unique in that it utilizes multiple beams, noncoplanar arcs of radiation to deliver the therapeutic dose. Figure 1 displays the effects of multiple beams on a mass when compared to single beam radiation. Diagram 1 is a graphic representation of a single beam of radiation. The area of Dmax in 1 clearly falls outside of the target area. Diagram 2 is a representation of multiple beams (A-F) which intersect at the target and the dose delivered to the target is a summation all the beams. Although the Dmax for each beam would still fall out of the target area, the dose delivered to the target would be in the order of 300% (6x50%) of the Dmax dose. SRS typically uses more beams (9) which would further enhance radiation delivery and spare normal tissue.
| 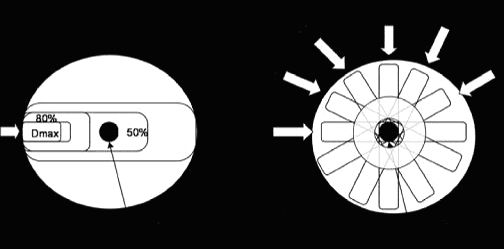 Figure 1. Diagrams 1 is a schematic representation of the radiation dose delivered to a target using a single beam of radiation and 2 represents multiple beams (A-F). The inner circles in 2 represent isodose lines. |
|
| |
Generally in planning SRS we start with 9 equally spaced 100 degree arcs per isocenter (center of the cancer or target). These arcs are spaced on average 20 degrees from each other. Apart from the multiple arcs (beams) a number of other planning tools can be used in SRS, these are; arc weighting and multiple isocenters. Multiple isocenters are used where the target has an irregular shape e.g., triangular shape.
DOSE SELECTION
In choosing a dose for SRS numerous factors play a role. First do no harm, select a radiation dose which is the lowest dose that is effective. Higher doses can sometimes give a more rapid response but often leads to unacceptable complications. Based on dose-volume relationships larger masses receive lower doses, this is because larger masses have exponentially more normal tissue exposed to radiation. The method used in selecting a radiation dose is based on the 1% (3%) isorisk line which is a logarithmic scale graph relating lesion diameter to 1% risk of complications (3). This logically leads to a restriction in the size of the mass that can be treated with a single dose of SRS. This is currently judged to be 4 cm in diameter, as masses larger than this would receive subtherapeutic doses of radiation. We currently use doses from 1250 to 2100 cGy depending on the mass. Unfortunately at this stage extrapolated doses from human SRS are being used.
RADIATION DELIVERY
All animals receive general anesthesia and are monitored for the duration of therapy. SRS uses a modified Linac (6 MV) and gantry, and the dogs with the biteplate array are positioned at the Linac isocenter, see Figure 2. Both the couch and gantry can move. In a previously described technique the dogs head was placed in a head frame which was fixed to couch of the CT and then with treatment fixed to the Linac couch (1). This allowed localization using immobilization of the head frame. The frameless stereotactic technique separates localization from immobilization and uses real-time tracking (4). Localization is achieved through detection of the four passive markers attached to the maxillary biteplate or bone attached array(fiducial) which forms a rigid system. This tracking is performed in real-time using a charge-coupled device (CCD) optical system equipped with infrared light which flood the room with infrared light (RadioCameras, Zmed, Hollistan, MA). The light is reflected of the four passive markers and these are then read by the CCD optics. Linking the position of the markers with the CT and a calibration matrix that relates the camera position to the Linac isocenter allows tracking of the target relative to the Linac isocenter. The system is calibrated regularly to ensure accuracy of planning. Typically each isocenter requires about 15-20 minutes treatment time. The localization accuracy of frameless SRS has been reported to be 1.1 ± 0.3 mm (4). Another collimation system is being tested currently called a multileaf collimator. This allows for dynamic adjustment of the collimator during radiation delivery.
| 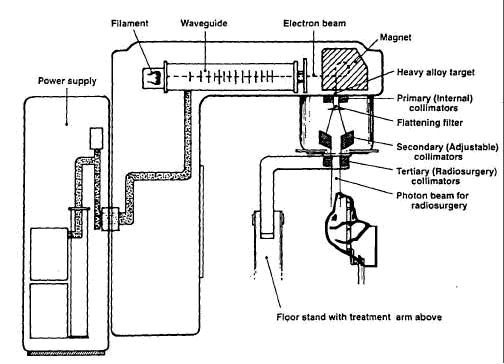 Figure 2. Linac with stereotactic radiosurgery gantry fitted (floor stand and tertiary collimators). Adapted from figure 6.1 in reference 3. |
|
| |
RESULTS
The College of Veterinary Medicine has treated 49 cases of which 15 were intracranial and the 34 extracranial. The extracranial tumors have been predominately nasal tumors followed by oral tumors, appendicular osteosarcomas and feline vaccine associated soft tissue sarcomas. Early radiation effects encountered have been; dermal changes at the site, such as mild erythema to acute moist dermatitis and ulceration. Acute ocular changes where applicable included transient to permanent decrease in lacrimation and mild keratoconjunctivitis. Mucositis was not a limiting factor when treating nasal or oral tumors. When it was present, it was mild. Late radiation effects at the radiation site included alopecia, mild ocular changes (no blindness), loss of hair color, hyperpigmentation. The current funded study at the College is in the follow-up phase and so survival comparisons cannot be made with conventional fractionated radiation at this stage. However, if results prove to be comparable the benefits to the patient and owner would include a single treatment session requiring less hospitalization time, and reduced complications (normal tissue sparing).
References
1. Lester NV, Hopkins AL, Bova FJ, Friedman WA, Buatti JM, Meeks SL et al. Radiosurgery using a stereotactic headframe system for irradiation of brain tumors in dogs. J Am Vet Med Assoc 2001; 219(11):1562-7.
2. (Buatti JM, Meeks SL, Friedman WA, Bova FJ. Stereotactic radiosurgery: techniques and clinical applications. Surg Oncol Clin N Am 2000; 9(3):469-87.
3. Friedman WA, Buatti JM, Bova FJ, Mendenhall WM. Linac Radiosurgery. A Practical Guide. Berlin: Springer, 1998.
4. Ryken TC, Meeks SL, Pennington EC, Hitchon P, Traynelis V, Mayr NA et al. Initial clinical experience with frameless stereotactic radiosurgery: analysis of accuracy and feasibility. Int J Radiat Oncol Biol Phys 2001; 51(4):1152-8.
5. Bouchet LG, Meeks SL, Goodchild G, Bova FJ, Buatti JM, Friedman WA. Calibration of three-dimensional ultrasound images for image-guided radiation therapy. Phys Med Biol 2001; 46(2):559-77.