Antioxidant Deficiencies in Hospitalized Dogs and Cats [State of the Art Lecture]
Lauren A. Trepanier, DVM, PhD, DACVIM, DACVCP
Reactive oxygen species (ROS) are molecular oxygen metabolites that are highly reactive with lipids, proteins, and DNA, causing oxidative damage to these cellular macromolecules. This damage, termed oxidative stress, accumulates over time and is thought to contribute to both disease pathology and the aging process. Cellular mechanisms that exist to counteract ROS include stabilization by enzymes such as superoxide dismutase and catalase, and direct scavenging by antioxidant molecules such as glutathione (GSH), a major intracellular antioxidant; cysteine, a precursor of GSH and a major extracellular antioxidant in plasma; vitamin E, a major lipid soluble antioxidant; and ascorbate, a critical intracellular and extracellular antioxidant.
Figure 1. | 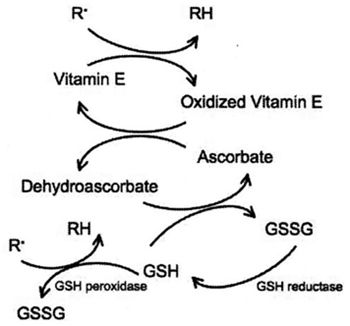 The inter-relationship between membrane bound vitamin E (a-tocopherol), ascorbate, and glutathione, in the scavenging of free radicals. |
|
| |
Oxidative Stress and Critical Illness
Oxidative stress is thought to play an important role in the pathogenesis of many diseases in humans, to include cardiovascular disease, chronic inflammatory disorders, and various cancers.1,2 ROS lead to direct oxidative tissue injury as well as to the activation of genes that perpetuate inflammation. ROS also deplete endogenous antioxidants, which may increase susceptibility to oxidative injury from the underlying disease or from some drug treatments. Deficiency of GSH, the major intracellular thiol, has been shown in human patients with acute pancreatitis, HIV infection, hyperthyroidism, Type I diabetes, and cirrhosis,3-6 and has been associated with disease severity and decreased survival in some studies.7,8 The amino acid cysteine, a component of glutathione, is rate-limiting for glutathione synthesis and itself acts as a direct antioxidant in plasma. Cysteine deficiency has been associated with conditions such as HIV infection in humans.9 Some studies in critically ill humans have actually shown an increase in plasma cysteine accompanying a decrease in intracellular glutathione,10,11 suggesting a defect in, or down-regulation of, glutathione synthesis from cysteine.
Vitamin E helps to prevent oxidative damage to cell membranes. Vitamin E levels have been shown to be deficient in human patients with diabetes, septic shock, hyperthyroidism, or ARDS.12-14 Ascorbate is essential for maintaining vitamin E in its reduced, active form. In addition, ascorbate constitutes the first line of defense against neutrophil-derived oxidants, and is capable of reducing methemoglobin to hemoglobin in plasma. Ascorbate is oxidized to dehydroascorbate in plasma, which is recycled back to ascorbate by GSH as well as by several enzyme systems in erythrocytes, neutrophils, endothelial cells and hepatocytes.15 Deficiencies in ascorbate have been reported in critically ill human patients with HIV infection, myocardial infarction, lymphoma, type II diabetes, sepsis, or renal failure.16-21 Ascorbate deficiency is associated with disease severity in acute pancreatitis,22 and is predictive of multiple organ failure in surgical patients.23 Low ascorbate has also associated with increases in blood pressure in humans.24 Interestingly, ascorbate spares glutathione, and has been shown to increase glutathione concentrations in human patients,25,26 even in those with hereditary defects in glutathione synthesis.27 Conversely, ascorbate deficiency has been associated with decreases in glutathione concentrations in humans.
Much less is known about antioxidant status in clinically ill dogs and cats. Serum vitamin E concentrations are decreased in dogs with immune-mediate hemolytic anemia,28 and hepatic glutathione concentrations are decreased in dogs and cats with various hepatic diseases.29 Some drug intoxications, classically acetaminophen, are known to deplete glutathione.30,31 We recently completed a study of antioxidant concentrations in dogs treated for various diseases at the Veterinary Medical Teaching Hospital at the University of Wisconsin-Madison.32 Overall, we found significantly decreased reduced glutathione concentrations (as measured in erythrocytes) in ill dogs (n = 61) compared to healthy dogs (n = 37), with significantly greater glutathione deficiency with increased severity of disease. Plasma cysteine and plasma ascorbate were unchanged in ill dogs. Importantly, glutathione deficiency was more pronounced in dogs that did not survive to discharge from the hospital, compared to survivors. Dr. Katrina Viviano at UW-Madison is now beginning a prospective study to determine whether glutathione supplementation (via N-acetylcysteine) has a beneficial effect on redox status, clinical illness score,33 and survival in these hospitalized dogs.
We have also completed a similar survey of glutathione, cysteine, and ascorbate concentrations in hospitalized cats, with surprising results. Ill cats with various diseases (n = 37) had no differences in plasma cysteine or erythrocyte reduced glutathione compared to healthy cats (n = 33).32 However, plasma ascorbate concentrations were significantly higher in ill cats compared to healthy cats. Increases in plasma ascorbate were even observed in FIV-infected cats, in contrast to ascorbate deficiencies that have been observed in HIV-infected humans. It is possible that cats either up-regulate ascorbate synthesis or decrease ascorbate break-down during illness. These results indicate that illness is associated with a substantially different antioxidant response in cats compared to dogs. The relationship between this response and both glutathione homeostasis and overall antioxidant capacity is not yet clear, and additional research is planned to investigate this further.
Antioxidants and Aging
Oxidative stress also appears to increase with age, and may play a role in the aging process itself. In vivo oxidative stress and biological aging appear to be positively correlated.34 In humans, age-associated decreases in reduced or total glutathione have been found in several studies;35-37 interestingly, humans that successfully survive to the age of 100 appear to have better antioxidant capacity compared to average geriatric patients.38 In rats, aging is associated with decreased capacity for glutathione synthesis.39 Male patients with Alzheimer's, an age-related disease, also have decreased capacity for glutathione synthesis.40 Interestingly, superoxide dismutase and catalase are increased in older humans, suggesting a compensatory response to oxidative stress.41
In dogs, relatively little work has been done on antioxidants and aging. One study in aged and young Labrador retrievers found no differences in antioxidant potential (plasma reducing power) between groups.42 In another study in beagles in a research setting, measures of oxidative stress (malondialdehyde or MDA) were higher in older dogs, along with apparently compensatory increases in both glutathione peroxidase and SOD activities.43 Despite this, these older dogs had a deficiency in glutathione that was more pronounced in males than females. Antioxidant supplementation, along with behavioral enrichment, has been shown to have a positive effect on cognitive function in geriatric dogs, over that seen with behavioral enrichment alone.44 This suggests a role for antioxidant deficiencies in cognitive impairment.
In our recent studies in hospitalized dogs and cats, we did not find a correlation between age and antioxidant status.32 However, the patients in this population had various confounding diseases. Therefore, we embarked on a study to examine both reduced glutathione and cysteine in young versus 'older' dogs. We hypothesized that aged dogs, although healthy, would have lower concentrations of these antioxidants. We recruited 26 healthy young dogs, defined as skeletally mature dogs between 1 and 3 years of age, and 35 healthy 'old' dogs, defined, based on longevity studies in dogs,45 as > 7 years of age for large breed dogs (> 34 kg); > 8 years of age for medium sized dogs (14 to 33 kg), and > 9 years of age for small dogs (< 14 kg).
Surprisingly, we found essentially identical concentrations of reduced glutathione and plasma cysteine concentrations between these two groups of dogs. These dogs were mostly neutered and were being fed a variety of quality commercial diets. While we cannot conclude from this study design that aging has no effect on antioxidant status in dogs, we can conclude that otherwise healthy older pet dogs do not have deficiencies in glutathione or cysteine compared to younger dogs. Therefore, these findings do not support the empirical use of antioxidant supplementation in older healthy dogs, beyond that which is provided in commercial foods. Additional work is needed to determine the interaction, if any, between age and antioxidant status in clinically ill dogs.
Acknowledgements
These studies were funded by grants from the Waltham Foundation and from Purina Nestle.
References
1. Loria CM, et al. Am J Clin Nutr, 2000. 72(1): 139-45.
2. Roth E, N Manhart, B Wessner, Curr Opin Clin Nutr Metab Care, 2004. 7(2): 161-8.
3. Abalovich M, et al. Clin Endocrinol (Oxf), 2003. 59(3): 321-7.
4. Dominguez C, et al. Diabetes Care, 1998. 21(10): 1736-42.
5. Rahman SH, et al. Gastroenterology, 2004. 126(5): 1312-22.
6. Yamauchi A, et al. Antioxid Redox Signal, 1999. 1(2): 245-53.
7. Fiorelli G, et al. Eur J Clin Invest, 2002. 32 Suppl 1: 21-7.
8. Herzenberg LA, et al. Proc Natl Acad Sci U S A, 1997. 94(5): 1967-72.
9. Naisbitt DJ, et al. AIDS Res Hum Retroviruses, 2000. 16(18): 1929-38.
10. Lang CA, et al. Exp Biol Med (Maywood), 2001. 226(9): 866-9.
11. Lyons J, et al. Crit Care Med, 2001. 29(4): 870-7.
12. Aliciguzel Y, et al. Free Radic Biol Med, 2001. 30(6): 665-70.
13. Metnitz PG, et al. Intensive Care Med, 1999. 25(2): 180-5.
14. Ogilvie AC, et al. Intensive Care Med, 1991. 17(1): 40-4.
15. Upston JM, et al. Biochem J, 1999. 342 (Pt 1): 49-56.
16. Abou-Seif MA, A Rabia, M Nasr, Clin Chem Lab Med, 2000. 38(8): 737-42.
17. Galley HF, MJ Davies, NR Webster, Free Radic Biol Med, 1996. 20(1): 139-43.
18. Locatelli F, et al. Nephrol Dial Transplant, 2003. 18(7): 1272-80.
19. Nyyssonen K, et al. Atherosclerosis, 1997. 130(1-2): 223-33.
20. Trepanier LA, et al. J Acquir Immune Defic Syndr, 2004. 36(5): 1041-50.
21. Van Campenhout A, et al. Diabetes Metab Res Rev, 2006. 22(6): 444-54.
22. Bonham MJ, et al. Br J Surg, 1999. 86(10): 1296-301.
23. Borrelli E, et al. Crit Care Med, 1996. 24(3): 392-7.
24. Block G, et al. Hypertension, 2001. 37(2): 261-7.
25. Johnston CS, CG Meyer, JC Srilakshmi. Am J Clin Nutr, 1993. 58(1): 103-5.
26. Lenton KJ, et al. Am J Clin Nutr, 2003. 77(1): 189-95.
27. Jain A, et al, J Pediatr, 1994. 124(2): 229-33.
28. Pesillo SA, LM Freeman, JE Rush. Am J Vet Res, 2004. 65(12): 1621-4.
29. Center SA, KL Warner, HN Erb. Am J Vet Res, 2002. 63(8): 1187-97.
30. Allison RW, et al. J Am Vet Med Assoc, 2000. 217(8): 1157-61.
31. Hill AS, et al. Am J Vet Res, 2001. 62(3): 370-4.
32. Viviano K, et al. Proceedings of the ACVIM Forum. 2007. Seattle.
33. King LG, et al. Am J Vet Res, 2001. 62(6): 948-54.
34. Mezzetti A, et al. J Am Geriatr Soc, 1996. 44(7): 823-7.
35. Lang CA, et al. J Lab Clin Med, 1992. 120(5): 720-5.
36. Lenton KJ, et al. Am J Clin Nutr, 2000. 71(5): 1194-200.
37. Samiec PS, et al. Free Radic Biol Med, 1998. 24(5): 699-704.
38. Paolisso G, et al. J Am Geriatr Soc, 1998. 46(7): 833-8.
39. Liu RM, DA Dickinson. Antioxid Redox Signal, 2003. 5(5): 529-36.
40. Liu H, et al. Ann N Y Acad Sci, 2004. 1019: 346-9.
41. Rizvi SI, et al., Mol Biotechnol, 2007. 37(1): 58-61.
42. Blount DG, et al. J Nutr, 2004. 134(8 Suppl): 2120S-2123S.
43. Vajdovich P, et al. Vet Res Commun, 1997. 21(7): 463-70.
44. Milgram NW, et al. Neurobiol Aging, 2005. 26(1): 77-90.
45. Patronek GJ, DJ Waters, LT Glickman. J Gerontol A Biol Sci Med Sci, 1997. 52(3): B171-8.