Molecular Analysis of Stress-Activated Proteins and Genes in Dolphins and Whales: A New Technique for Monitoring Environmental Stress
Abstract
In the past several decades, there has been a worldwide increase in marine diseases resulting in mass mortality among all major taxa and shifts in ecologic community structures in the oceans.1 Marine mammals have experienced a pandemic of morbilliviral infections and outbreaks of diseases caused by influenza viruses, fungi and algal toxins. Many of the disease outbreaks appear to have been facilitated by increased environmental stress burden in the global marine ecosystems due to changing environmental conditions triggered by climate variability and human activities. It is imperative to develop novel health-monitoring tools that could guide the management of marine ecosystems and facilitate the conservation of key species. Our research is focused on the molecular mechanisms underlying molecular stress response in humans and cetaceans exposed to environmental stress and disease. We have developed new techniques for detecting the molecular signature of stress based on molecular analysis of stress-activated proteins and genes in field tissue specimens.2 The detection of molecular stress signature has been applied to evaluate the impact of tuna fishery on the spotted dolphins in the Eastern Tropical Pacific, the effects of coastal pollution on the beluga whales in the St. Lawrence River, and the idiopathic population decline of the North Atlantic right whale population.
Introduction
What is the general biologic impact of diverse stressors on organisms? Recent findings suggest that the common outcome of environmental stress and disease is perturbed homeostasis and triggering of cellular stress. Cellular stress represents unstable states of cellular existence characterized by altered redox balance, DNA/protein damage and high energy expenditure.1-5 Cellular stress in turn triggers molecular stress response (MSR), a homeostasis-restoring process that has evolved in all living organisms.3,8-13 MSR is triggered in early phases of the health-perturbation process (symptom-free phase, within hours after perturbation) and persists until recovery.11-15 In mammals, MSR recruits many fundamental intracellular and systemic processes including cellular detoxification and oxidative stress defense, DNA/protein repair, cell cycle regulation, programmed cell death (apoptosis), cell adhesion, and responses by the endocrine, immune and nervous systems. MSR is necessary but when prolonged it burdens the organism and instead of restoring health it leads to disease and further MSR amplification through a variety of mechanisms including microbial infections, carcinogenesis, autoimmune disorders, neurodegeneration, and accelerated aging amongst others. In human medicine, the importance of MSR in the physiology and pathogenesis of many diseases has been extensively documented for a broad range of stressors.16-23 In summary, MSR is the common response to diverse stressors that affect health.
We have suggested that MSR could be detected using the expression patterns of multiple stress-activated proteins and genes as a complex molecular stress signature.2 Recognition of the signature would indicate the presence of MSR thus signaling compromised health status. This is similar to the detection of the presence of a broad range of dangerous/non-self molecules (antigens) by the mammalian immune system. Detection of dangerous stressors using MSR signature has the advantage that no previous knowledge of the encountered stressor is needed. This is important in detecting the impact of previously unrecognized/emerging stressors. Recognition of MSR in stress-sensitive species could serve as an early warning of compromised health and a guide for monitoring the impact of conservation/management strategies on the stability of ecosystems. The molecular components of stress response are highly conserved among mammals, allowing a common diagnostic protocol for all cetacean species. Cellular stress is rapidly spread to many tissues in addition to those affected by the primary stressor (amplification through second messengers), implying that MSR detection could be based on tissues practical for repeated sampling such as skin or blood.
Methods
Skin specimens preserved in DMSO/NaCl or formalin, or frozen, were obtained from normal and stressed dolphins and whales. Different cetacean species were analyzed: the bottlenose dolphin (Tursiops truncatus), the common dolphin (Delphinus delphis), the spotted dolphin (Stenella attenuata), the spinner dolphin (Stenella longirostris), the beluga whale (Delphinapterus leucas), the right whale (Eubalena glacialis), and the grey whale (Eschrichtius robustus). To calibrate the stress signature analysis, we have assembled a control group of samples representing wild and semi-domesticated animals with known exposure to stress. The negative controls were normal healthy animals. The positive controls experienced various clinically diagnosed diseases or trapping in polynya (a closing polar ice hole). Stress-activated proteins were visualized using immunohistochemical staining of thin sections as described previously.2,24 The expression levels were determined using light microscopy and image analysis. Stress-activated genes were identified using GeneFilter analysis (Research Genetics) and HAPI software in collaboration with the UCSD Genomics Core Laboratory.
Results and Discussion
Molecular Stress Signature Based on Stress-activated Proteins
We have identified a group of over 30 stress-activated proteins (SAP) whose expression pattern is significantly altered in skin and blood of stressed cetaceans but not in the normal animals. The SAP are structurally and functionally diverse molecules with recognized roles in oxidative stress response, cellular detoxification, cell growth and differentiation, programmed cell death (apoptosis), immunologic, hormonal and neurologic signaling and cell adhesion (Table 1). The general pattern of SAP induction is conserved in different cetacean species, genders, ages, and in broad range of stressors. We have developed a technique for rapid and sensitive detection of multiple stress-activated proteins by immunohistochemical staining and image analysis using a multi-target SAP antibody that can detect multiple SAP simultaneously. SAP can be visualized in typically preserved field specimens (DMSO, formalin, ethanol) or frozen tissues. This technique allows screening large numbers of specimens, as necessary for ecologic studies, to correlate the presence of cellular stress with various environmental and anthropogenic factors such as coastal pollution or tuna fishery.
Molecular Stress Signature Based on Stress-activated Genes
We have identified over 30 genes whose expression is upregulated by stress in cetacean skin. These stress-activated genes were discovered by panoramic analysis of gene expression in normal and stressed belugas using human DNA chips (gene microarrays). This approach takes advantage of our finding that, under certain hybridization conditions, commercially available human gene microarrays can be applied for cetaceans. Gene-expression analysis requires the use of snap-frozen (or directly solubilized in Trizol) specimens, to preserve RNA. A panel of 30-50 stress-activated genes will be used to design a custom DNA stress chip to allow more efficient analysis of large number of samples.
As a long-term goal, we envision detection and interpretation of SAP/SAG based molecular stress signatures using automated detecting devices coupled with a dedicated computer-assisted information system. The multi-marker molecular stress signatures could be employed not only for mammals but, in principle, also for other animals and plants. Multi-species analysis could potentially provide better insight into the multi-faceted mechanism by which environmental stress affects ecosystems.
Table 1. Stress-activated proteins in human patients and cetaceans.a | 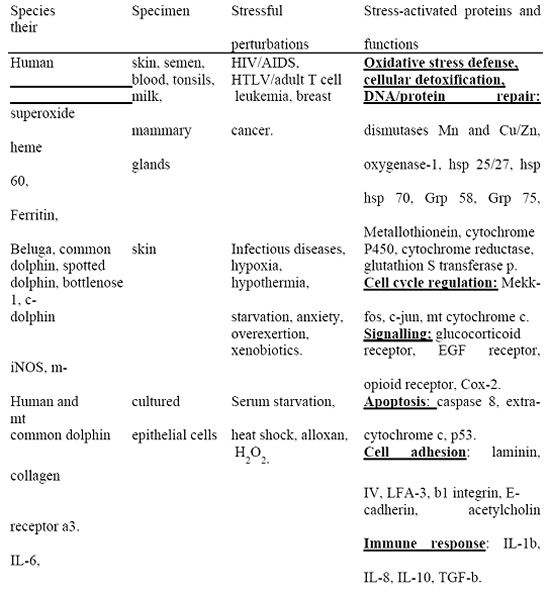
|
|
| |
a. The information in this table is based on our unpublished results.
Acknowledgments
This work was supported by the International Dolphin Conservation Program Act of 1997. S. Southern is a Senior NRC Fellow.
References
1. Harvell CD, Kim K, et al. 1999. Emerging marine diseases-climate links and anthropogenic factors. Science 285:1505-1510.
2. Southern SO, A Dizon. 1999. Molecular analysis of stress response in dolphins and whales: a new technique for monitoring environmental stress. 13th Biennial Conference of the Marine Mammal Society. 11/28-12/3, Maui, Hawaii.
3. Feder ME. 1999. Organismal, ecological and evolutionary aspects of heat-shock proteins and the stress response. Am. Zoologist 39:857-864.
4. Burton GW, KU Ingold. 1984. Beta-carotene: an unusual type of lipid antioxidant. Science 224: 569-573.
5. Halliwell B, JMC Gutteridge. 1985. Oxygen radicals and the nervous system. Trends Neurosci. 8:22-26.
6. Breimer LH. 1990. Molecular mechanisms of oxygen radical carcinogenesis and mutagenesis: the role of DNA base damage. Mol. Carcinogen 3:188-197.
7. Gregus Z, CD Klaassen. 1996. Mechanisms of toxicity. In Casarett and Doull's Toxicology: the Basic Science of Poisons, 5th edition. C.D. Klaassen (ed.), New York: McGraw-Hill. pp.35-74.
8. Von Schantz T, S Bensch, M Grahn, D Hasselquist, H Wittzell. 1999. Good genes, oxidative stress and condition-dependent sexual signals. Proc. Royal Soc. Lond. Br. Biol. Sci. 266:1-12 .
9. Iwama GK, MM Vijayn. 1999. Heat shock proteins and physiological stress in fish. Am. Zoologist 39: 901-909.
10. Becker J, EA Craig. 1994. Heat shock proteins as molecular chaperones. Eur. J. Biochem. 219:11-23.
11. Tibbles LA, JR Woodgett. 1999. The stress-activated protein kinase pathways. Cell Mol. Life Sci. 55(10):1230-54. Review.
12. Buchmeier NA, F Heffron. 1990. Induction of Salmonella stress proteins upon infection of macrophages. Science 248:730-732.
13. Zügel U, SH Kaufmann. 1999. Role of heat shock proteins in protection from and pathogenesis of infectious diseases. Clin. Microbiol. Rev. 12(1):19-39.
14. Tyrell RM. 1996. UV activation of mammalian stress proteins. In: Stress-Inducible Cellular Response. U. Feige, R.I. Morimoto, I. Yahara and B. Polla (eds.), Birkhäuser Verlag Basel/Switzerland. Pp. 255-271.
15. Shohami E, I Gati, E Beit-Yannai, V Trembovler, R Kohen. 1999. Closed head injury in the rat induces whole body oxidative stress: overall reducing antioxidant profile. J Neurotrauma. 16(5):365-76.
16. Piedimonte G, D Guetard, M Magnani, D Corsi, I Picerno, PSpataro, L. Kramer, M Montroni, G Silvestri, JF Torres Roca, L Montagnier. 1997. Oxidative protein damage and degradation in lymphocytes from patients infected with human immunodeficiency virus. J. Infect. Dis. 176(3):655-64.
17. Bashir S, G Harris, MA Denman, DR Blake, PG Winyard. 1993. Oxidative DNA damage and cellular sensitivity to oxidative stress in human autoimmune diseases. Ann. Rheum. Dis. 52(9):659-66.
18. Stanek D, G Koaecká, M Oltman, A Keleová, E Jahnová. 1995. Links between prolonged exposure to xenobiotics, increased incidence of hepatopathies, immunological disturbances and exacerbation of latent Epstein-Barr virus infections. Int. J. Immunopharmacol. 17(4):321-8.
19. Terekhina NA, IuA Petrovich, et al. 1998. Lacrimal and salivary antioxidants in viral infection. Klin. Lab. Diag. 1: 13-15.
20. Spector A. 1995. Oxidative stress-induced cataract: mechanism of action. FASEB J. 9(12):1173-82. Review.
21. Barnes PJ. 1995. Nitric oxide and asthma. Res. Immunol. 146:698-702.
22. Narayanan PK, KE LaRue, EH Goodwin, BE Lehnert. 1999. Alpha particles induce the production of interleukin-8 by human cells. Radiat. Res. 152(1):57-63.
23. Sanocka D, R Miesel, P Jedrzejczak, MK Kurpisz. 1996. Oxidative stress and male infertility. J Androl. 17(4):449-54.
24. Southern SO, PJ Southern. 1998. Persistent HTLV-I infection of breast luminal epithelial cells: a role in HTLV-I transmission? Virology 241:200-215.